In early 2010, I conducted research into fusion power as part of my final year of the physics honours program. I spent several months reviewing literature on the subject. This cumulated in compiling a comprehensive introduction to the topic as well as delivering a one-hour talk on the subject three times.
My presentation slides are freely available (in ppt or pdf formats) for those who are interested in presenting on this topic themselves. If you want to use my content, please mention where you got it.
This is a series of posts is based on the research I conducted at that time. These posts are intended to provide a general introduction to the field of nuclear fusion. This article gives the overview of the major topics. Subsequent posts will expand in more detail on these subjects.
If someone is interested in digging into the underlying physics of fusion power, I have laid out some resources in the science behind fusion power. There are also a number of useful tools for understanding fusion power.
Contents
What is Nuclear Fusion?
Nuclear fusion refers to any process of interaction of two nuclei in which they combine to form a heavier nucleus. For light elements, this process typically emits particles such as other nuclei and neutrons along with a relatively large amount of energy.
The reason we are interested in nuclear fusion (for power production) is that these reactions can release much more energy than is required to make them happen. A very simple analogy would be the combustion of wood. Energy is required to raise the heat of the wood high enough for it to ignite. Once we have it ignited however, it produces enough energy to keep itself burning and also release a lot as heat.
Clarifying fission and fusion
The most stable elements on the periodic table are iron and nickel1 . Nuclear fusion reactions tend to release energy if they combine light elements into heavier products (as long as the products are lighter than iron/nickel).
On the flip side, nuclear fission reactions that split very heavy nuclei into several products, releasing energy. If you are interested in nuclear fission power, we have written a large article on how nuclear fission works as well as a series of posts on nuclear myth and fact.
One can imagine fusion as building heavier atoms, and fission as breaking up very heavy atoms. Each process liberates energy that is stored in the bonds between nucleons (protons and neutrons).
Fusion as a power source
The goal of fusion power production is to harness reactions of this nature to produce electrical power. In the interests of creating reliable power, it is most desirable to have an energy source that is steady and predictable, rather than intermittent.
Energy Capture
Emitted energy from the commonly proposed fusion reactions is primarily in the form of high-energy neutrons and various charged particles.
- Charged particles skid to a halt mainly through electromagnetic interactions.
- Neutrons deposit energy primarily through nuclear interactions.
Stopping neutrons generally requires different shielding than charged particles. Neutrons cannot be ‘steered’ onto collection areas because they have no electric charge. The heating created by neutron radiation will be in all directions. This means that shielding will be very differently designed depending on the fuel cycle. The fusion fuel cycles, which we look at in more detail later on, are the different reactions that light elements can undergo. A typical fusion reaction involves reactants that interact to create products, releasing energy.
We more deeply at these concepts in another of our posts on how we turn nuclear fusion power into electricity.
Thermal Conversion
Using fusion as an energy source, we can produce heat. Thermal power plants convert heat into electricity via a heat engine. A well-known example of a heat engine is the steam turbine, which produces around 80% of the world’s electricity. Thermal engines are a tried and true method for creating electricity. Most proposed fusion techniques involve a thermal power plant design.
Converting heat to electricity is generally around 33-50% efficient. That is, we are able to convert 33-50% of the heat energy into electricity. Heat engines rely on a difference in temperature between two thermal reservoirs. The efficiency with which we can convert heat into electricity is governed by a concept called the Carnot efficiency. The Carnot engine is a theoretical ‘ideal’ heat engine, which does not increase the level of entropy in the universe. It is impossible to construct a heat engine that is more efficient than the Carnot engine. Such an engine would violate the second law of thermodynamics and would be able to create infinite energy.
Our large thermal power plants are able to convert heat into electricity with an efficiency that is rather close to the Carnot efficiency. We can improve the Carnot efficiency by having the two heat reservoirs at very different temperatures. The more different the relative temperatures are, the greater the Carnot efficiency will be. Also, our working fluid has some effect on the maximum possible efficiency. Steam engines tend to top out at around 600ºC, while brayton-cycle gas turbines can go above 1000ºC.2
Direct Conversion
Direct conversion involves capturing charged particles to create a current. In this case the energy is never captured intentionally as heat. These systems are designed to instead create a current directly. Direct conversion has been considered for some reactor types that may be built in the future. The reason we want direct conversion is because it may be possible to achieve efficiencies of 90% in the conversion of fusion power to electrical power.
In order to utilize direct conversion systems effectively, we need to be using a fusion process that produces only a very small number of neutrons. This is because neutrons, lacking electric charge, cannot be ‘steered’ onto certain collection plates. We will discuss this in much more detail in a later post.
These low-neutron processes do exist. The main problem is that these processes require higher energies (temperatures) than some other processes. The engineering challenges of reaching these higher energies are significant, and may not be met for a very long time. However, there are some intriguing concepts being presented that may make direct conversion feasible in the coming decades. We will discuss direct conversion in much more detail in a later post.
Update: We included an in-depth look at direct conversion in a later post entitled how do we turn nuclear fusion power into electricity?
Net Energy
We want net energy output from our fusion power plant. A fusion power plant must produce more energy than the energy required to run the plant’s machinery. So we need to liberate more energy from fusion reactions than it takes to create the conditions under which they happen.
Humans have demonstrated that fusion is possible many times. Devices such as the fusor are even relatively easy to build. However, it is very difficult to create a fusion system that can actually produce more energy than it takes to run. We know that it is possible, but it is proving to be very difficult to turn experimental results and knowledge into a functioning power system.
In a later post we will look at the details of the fusion energy gain factor Q, a useful quantity for describing the energy balance of a reactor. Q is a letter used often in various areas of physics, has special meaning in considerations of fusion power plants.
Steady-state power
In order to be producing baseload electrical power, the reaction must be either in dynamic equilibrium or pulsed quickly. The goal of fusion power systems is thus to create ‘steady state’ power, where the net output of the power plant is constant. What have we accomplished so far with regards to achieving steady state operation?
JET (1982-present) (Joint European Torus) – 20-60 second pulses are possible, but high energy ones are less than a second.
ITER (~2018) (originally International Thermonuclear Experimental Reactor) – ITER is expected to be able to produce 500MW for up to 1000 seconds.
DEMO (~2033) (DEMOnstration Power Plant) – Hopefully steady state achievement around 2033, add electricity to grid around 2040.
Safety Concerns
The most popular fusion reactions produce a lot of neutron radiation. For instance, the most popular fuel cycle for future reactor designs Deuterium-Tritium releases about 80% of its energy in the form of high energy neutrons.
‘Flux’ is a term that means flow or discharge. When we discuss ‘neutron flux’, we are discussing the flow of neutrons out from the reactor. In other contexts, one might discuss ‘heat flux’, which would be the flow of heat. For example, a large and hot camp fire creates a greater heat flux than a small one.
This fact has associated safety concerns:
- Direct Neutron Flux – We need to deal with the direct effects of the flux on our people, equipment and electronics.
- Activated Materials – We need to consider the human exposure to activated materials as well. Activated materials are sometimes created when a neutron is captured by a stable nucleus. The captured neutron changes the isotope of the nucleus. If the new isotope is radioactive, it has been ‘activated’. Some activated materials undergo changes in their chemical properties. These changes would have to be planned for in the initial design. Much of humanity’s experience in this area has been gained from the neutron fluxes from fission reactors. We look at this topic in more detail in our post about fusion reactor design.
Pieces of the puzzle
Most of the scientific work in fusion has been focused on achieving net energy gain. Without net energy gain, we cannot harness nuclear fusion as an energy source. We have not yet succeeded in achieving net energy gain. This is really the fundamental facet to fusion power research, if we cannot do this, then there isn’t much point to talking about nuclear fusion as a terrestrial energy source.
Fusion for power production requires:
- Fusion fuel cycles – the specific reaction we are trying to create. Reactants are usually very light elements. They combine under certain conditions, releasing energy and product isotopes.
- Fusion technique – a way of bringing the fuel physically into a state in which fusion can progress. This is our physical system for achieving fusion. Examples of this are the tokamak, laser implosion fusion, fast-ignition fusion, and the Polywell design. We have also written an introduction to the difficulties of designing a fusion reactor.
Fusion Fuel Cycles
Fusion fuel cycles are the possible fusion reactions. In order to be possible fusion reactions, the process must abide by the rules of nuclear physics, and yield net energy. Many fusion reactions are possible within these criteria, but only a few seem to be of use for fusion reactors on the earth. We will dig into the reasons for this in a later post.
Fusion reactions are analogous in concept and notation to chemical reactions. For example: The Deuterium-Tritium reaction is written as:
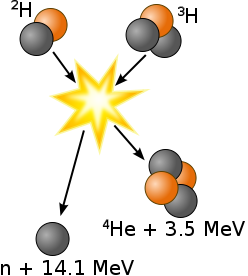
We can see that a deuterium and a tritium fuse to produce a helium-4 nucleus and a neutron. The 4He and the neutron carry 3.5 MeV and 14.1 MeV of kinetic energy respectively. That is, these particles are moving extremely fast away from each other after the fusion occurs. Never seen the “eV” unit before? It is an electron volt, a unit of energy that is very useful when studying nuclear interactions.
This is the D-T reaction, which has seen the most extensive research in the last few decades. Using the masses of these isotopes, and conservation of energy and momentum, we can determine the energy released and the distribution of momentum and energy between these two products.
For a deeper look, see our post on what fusion fuel cycles are and how they work.
Fusion Techniques
These are the different physical methods of achieving fusion conditions. They are the ways of achieving conditions under which fusion occurs at a rate and in such a manner as to be useful.
Nuclei require kinetic energy to overcome the Coulomb barrier between them. The coulomb barrier is the term used to describe the tendency of positively charged nuclei to repel each other.
Once the nuclei are close enough to each other, the strong nuclear force becomes stronger than the electrostatic force, and the nuclei may fuse. We say ‘may fuse’ because fusion is just one of several things that can happen when the nuclei interact. For instance, elastic and inelastic scattering interactions are also possible.
Some techniques we look at later include laser implosion, inertial electrostatic confinement (including the Polywell project), and the tokamak.
Stay tuned
This is the first post in a series on nuclear fusion power. Stay tuned for more information about this challenging research and engineering topic.
Posts in this series:
- The science behind fusion power.
- Helpful tools for understanding nuclear fusion.
- Fusion fuel cycles: What they are and how they work.
- The basic realities of fusion reactor design.
- How do we turn nuclear fusion power into electricity?
- Laser implosion fusion reactors
- Fusion fast ignition systems
- The binding energy per nucleon is the highest for iron/nickel. Therefore it isn’t feasible to extract energy from either fission or fusion of iron and nickel. A useful resource on the subject is the Wikipedia page for Nuclear binding energy. Accessed November 5th, 2010. [↩]
- Forsberg, C., Peterson, P., Zhao, H. (2007) High-Temperature Liquid-Fluoride-Salt Closed-Brayton-Cycle Solar Power Towers. Journal of Solar Energy Engineering, Vol 129, 141. [↩]
We have achieved net energy gain
Can a pressure wave with enough density can be used as a means to steer a large mass of neutrons in nuclear reactions?